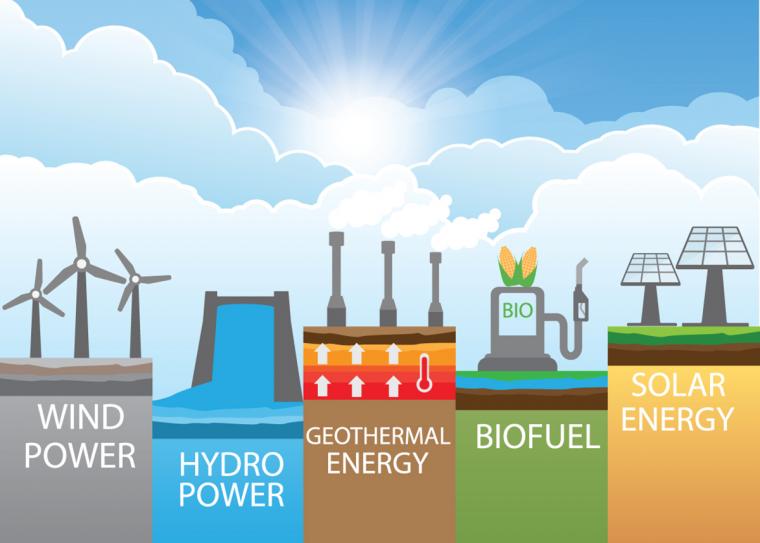
By the end of today, every one of us will have likely contributed to the global carbon footprint. Whether we rode in a car, used electricity, ate a cooked meal or used heating or air conditioning, at some point carbon-fueled devices were used to power our day. We are one of 7.5 billion people on Earth, and by 2060, we’re one of 10 billion. Just as the people of Easter Island eventually overexploited their resources, our question becomes, “What can we do to be sure we don’t overexploit ours”?
One way is to further develop renewable energy; we owe it to our future.
Today, more people on our planet have been born “on the grid” than off. According to recent World Bank and International Energy Agency statistics, nearly 88 percent of world population has access to electricity. Demand for energy is being driven not just by manufacturing, international trade, distribution and other industrial applications, but also by a growing population that depends on reliable streams (which currently is much less than 88 percent) of electricity to meet the requirements of daily life.
Data from the U.S. Energy Information Administration (EIA) supports these projections. According to the organization’s International Energy Outlook 2017, world energy consumption will grow 28 percent in 22 years, with most of this increase coming from countries that are not in the Organization for Economic Cooperation and Development (OECD). Non-OECD Asia (which includes China and India) is expected to account for over 60 percent of this growth.
Given finite reserves of petroleum, coal and other traditional energy sources, meeting the world’s rapidly increasing, long-term demand for electricity will require greater focus on reliable sources of renewables. Already, the EIA estimates that year-over-year growth in renewable energy consumption will average 2.3 percent per year through 2040. To meet this demand, governments, universities and research institutions around the world are partnering with private investors, foundations and individual donors to develop solutions to meet both the demand requirements, to address environmental concerns and the eventual scarcity of non-renewable resources.
In the United States, one example of this type of partnership is the University of Louisville’s Conn Center for Renewable Energy Research. In response to the challenge to lead Kentucky’s efforts in this area, the university established the Conn Center at the J.B. Speed School of Engineering in 2009 with the support of alumni benefactors Hank and Rebecca Conn. The Conn Center provides leadership and expertise, maintains world-class research facilities in renewable energy and promotes technologies, practices and programs that increase efficiency and efficacy for energy utilization worldwide. The Center also places a strong emphasis on partnering with key industry players, from suppliers of feedstock to energy end users, to focus on solutions that can be commercialized, therefore enabling real-world change.
Finding Solutions that Meet Population Needs
Such solutions — in the United States and worldwide — must take into account a number of key factors that are driving the development and deployment of energy from renewable sources. First, much of the demand will come from the developing world due in large part to rapid population growth. (In developed nations, there is a concurrent call to meet the energy requirements of rural and more isolated areas.) However, the economies of these countries often have limited resources available to fund massive investments in energy infrastructure. With this in mind, renewable energy solutions must be grounded in economic realities.
Second, energy generation must be accompanied by effective, reliable energy distribution — the two go hand-in-hand. While many areas of the world are technically on the grid, a large percentage of them lack a reliable electric supply. Current conditions in Puerto Rico, nearly a year after the devastating Atlantic hurricane season of 2017, demonstrate that large swathes of energy infrastructure in the developed world are also highly vulnerable to natural and man-made disasters. Depending on the sophistication and hardiness of the infrastructure, rebounding from such events can take years.
Third, energy technology is evolving at an exponential pace. Every month, it seems new breakthroughs in energy production, storage and distribution are being announced. At some point, however, these solutions must make it out of the laboratory and into commercialization. In an environment in which we are accustomed to a better electronic device being released every few months, it is difficult to make large-scale decisions between waiting for better or good enough, especially when millions of people and thousands of square miles may come into play.
Finally, choosing between macro grid and micro grid models can be difficult. Traditionally, our energy infrastructure has been built on the macro grid, which connects homes, businesses, public buildings and industrial facilities to a centralized power source. Conversely, the U.S. Department of Energy defines a micro grid as a group of interconnected loads and distributed energy resources within clearly defined electrical boundaries. A micro grid can connect and disconnect from the traditional macro grid, enabling it to operate in either grid-connected or “island mode,” even when the broader grid has become compromised, whether through unforeseen accidents or man-made events such as cyberattacks.
Micro grids offer greater local control, increased flexibility, price stability, reduced power loss (due to electricity traveling long distance over power lines) and a more consistent power supply. Equally important, they support the use of renewables, such as solar photovoltaic panels, biomass and other sources for storage of surplus power.
Using Puerto Rico again as our example, we have seen how an entire energy network can be compromised simultaneously. To restore power quickly and cost-effectively while the broader grid is being rebuilt, the U.S. Army Corps of Engineers along with companies including AES Energy Storage, Sonnen, Blue Planet Energy and Pura Energia and non-government organizations (NGOs) such as the Solar Foundation have deployed micro grid technology to bring power to some of the island’s hardest-hit, least-accessible areas. As the macro grid is restored, these relatively small systems can be relocated as needed or they can remain in place to provide a steady supply of electricity to local communities. Later, they can also contribute excess power to be fed into to the broader grid or into storage units.
Energy Storage: The Holy Grail of Renewables
One of the most challenging and exciting issues surrounding renewable energy is the question of storage. If excess electricity created within a micro grid during periods of low demand can be stored and made available during periods of high demand, the result is a more reliable flow of energy at lower cost.
According to researchers at the Conn Center, today’s leading electrochemical storage devices (including Li-ion, Li-S, Li-air and CFx batteries and super capacitors) cannot yet meet the required demand for transportation and commercial residential applications. Fundamental, basic scientific research must continue to develop long-lasting, rechargeable batteries. Nanomaterials-based electrodes, novel ionic and polymer electrolytes, advanced membranes and other basic materials, as well as rapid screening of materials for batteries and modeling of battery performance at the molecular level, are required to create the electrochemical storage systems to address future energy storage requirements.
Storage cost is as important as storage capability. Current battery technology is far below its theoretical energy density limit as today’s storage costs hover around $550 per kilowatt hour (kwh). As battery pack energy density improves through the use of new high-capacity cathodes, higher-voltage electrolytes and use of high-capacity silicon or tin-based nanostructured anodes — and even further through “beyond Li-ion” battery chemistries — battery costs should decrease significantly along with increased energy density storage.
A major factor driving battery storage costs is materials. Supplies of lithium and cobalt, two primary components of Li-ion batteries, are limited, located in countries that are somewhat politically unstable and somewhat toxic. Despite these downsides, current production levels need to be upscaled dramatically to meet future demand.
To justify the costs of production, batteries must also be able to cycle reliably through thousands of rounds of charge and discharge while being exposed to the harsh environment within a battery cell. Interphases and reactions between organic electrolytes and electrode surfaces must be stable and durable.
Today, significant research is being conducted globally in areas such as coatings, advanced manufacturing techniques, novel nanostructured electrodes and more, all with an eye toward creating batteries that are more reliable with increased cycles. Researchers are also aggressively pursuing the use of abundant, low-cost and less toxic materials such as aluminum, calcium, magnesium and zinc.
Collectively, this promising research should pave the way for more durable, reliable and energy dense batteries and electricity storage solutions soon.
Biomass: Fueling Economic Growth
In addition to their research into more efficient and reliable energy storage, the Conn Center and other institutions have also focused on developing technologies to convert biomass to useful fuels and chemicals. Just as electricity is needed to power the demands of daily life for people worldwide, fuels such as gasoline, diesel and jet fuel are needed to supply the energy demands of industry. This can involve heavy manufacturing in large factories; air, rail or marine shipping and distribution and/or light production in small enterprises.
Biomass raw materials can be divided into two primary categories: lignocelluloses containing carbohydrates, including wood, switchgrass, leaves, rice and wheat husks and lipids that primarily contain triglyceride esters, such as vegetable and animal fats and greases, waste oil and algae oils, among others.
Lignocelluloses generally lend themselves to the production of most types of fuels, while lipid triglyceride esters, because of the presence of long-chain fatty acids, are better suited to the creation of biodiesels and bio jet fuels.
While raw materials are in large supply, researchers work hard to address a number of challenges that impede full development of biomass as a fuel source. These include technical challenges such as the difficulty of fully breaking down and converting the raw cellulose substrate into simple, fermentable sugars and working with materials containing high solids content to maximize product concentrations. There are other concerns, such as minimizing water and energy inputs, creating fuels that can “drop into” the existing petrochemical industry infrastructure and the cost of reactors and other capital-intensive production facilities.
One concern of researchers has been the development of biofuels from nonedible feed stocks including nonfood energy crops, as well as agricultural residue, municipal solid waste and industrial waste. These materials are being used in second-generation biorefineries, designed to replace first-generation biorefineries that relied heavily on crops such as corn, wheat, barley, rye, cassava, sugar cane, etc. Since global demand for energy is tied directly to increased population growth, particularly in developing countries, it makes sense to avoid using raw material crops that could go toward food.
Have We Reached the Renewables Tipping Point?
The answer to that question in two words: not yet. Virtually every indicator makes it clear that renewables must play a greater role in meeting the global energy demands of an increasingly technology-dependent world. And although it would be premature to say that we are in a footrace against a rapid decline in traditional energy sources, it is clear that supplies of petroleum and coal will not last forever.
If nothing else, the goal today is to make sure research into the cost-effective development and distribution of energy from renewable sources continues. The Conns’ investment at the University of Louisville ensures that goal, one that Hank and Rebecca openly encourage others to fund. Said Hank, “While we are some years (or decades) away from depending fully on renewable energies, we, and the world we leave behind, cannot afford — at any level — to be caught unprepared.” T&ID